A magazine where the digital world meets the real world.
On the web
- Home
- Browse by date
- Browse by topic
- Enter the maze
- Follow our blog
- Follow us on Twitter
- Resources for teachers
- Subscribe
In print
What is cs4fn?
- About us
- Contact us
- Partners
- Privacy and cookies
- Copyright and contributions
- Links to other fun sites
- Complete our questionnaire, give us feedback
Search:
Under pressure
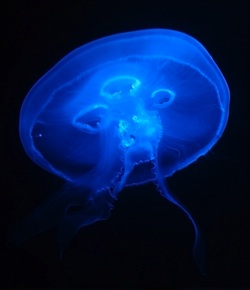
What have a jellyfish and some computer science techniques developed to allow computers to ‘see’, got to do with helping people recover from dance or football injuries? Bushra Akhtar, a computer scientist and biologist at Queen Mary, University of London is working on it!
You are made up of bits, little biological bits, called cells. When your cells work the way they should it’s great and you’re healthy, but in some medical conditions your cells undergo a change that stops them working the way they should. An example is with cells you use every day walking to school, dancing, playing football or riding a bike. These are the cells in your cartilage, and they have a tough, high-pressure job to do. Cartilage cells live in your joints: the joints of your arms, your legs, everywhere. Every step you take, your cartilage squashes and so do the cartilage cells inside the tissue. The cells detect these ‘mechanical signals’ and respond by producing all the things that keep the cartilage tissue healthy and strong. If this process goes wrong due to disease or if you stop squashing your cartilage (like weightless astronauts in space) then your cartilage breaks down.
The study of the way cells change shape when they are pushed, prodded or squashed, together with the biological consequences, is called ‘mechanobiology’. It’s a fascinating subject that involves understanding how all the clever engineering and physics inside a cell work, letting it keep its proper shape and perform its biological function. Examining the cells’ ‘mechanotransduction’ (how cells ‘sense and respond’ to mechanical forces like pressure) allows us to understand what goes wrong, and with that knowledge we can start to develop new medical treatments to help those who suffer these debilitating diseases.
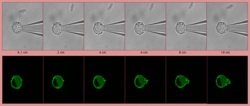
Let’s have a look
One of the ways researchers are trying to understand how cartilage cells work is by looking at how they respond to being squashed. A clever technique called ‘confocal microscopy’ is often used to get a picture of what’s happening in these tiny cells. In a normal microscope you flood the slide under the microscope with light and have a look at what bounces back, magnified through the lenses. This conventional way to look at cells had been very useful in medicine and biology. The problem, though, is that you see it all from one particular viewpoint. Cells are actually 3D, so it would be useful to be able to see the detail in a slice through them. Enter confocal microscopy. It uses the laws of optics, and a tiny pinhole, to remove the light that’s coming from anything other than the depth you are interested in. In effect it lets you see the information from a slice through your big and bulky cell. Using this method you can see what’s happening inside a cell at some particular level. Like a lift moving up through an office block, you can select the level in the cell to look at the information from. What’s more you can put all those slices together to make a 3D image that you can view from any angle.
It’s all about labels and jellyfish
Clever as it is, confocal microscopy can’t see the invisible. If you are interested in what gives a cell its mechanical properties, you have a problem. The cytoskeleton (the ‘skeleton of the cell’) is what gives the cell its shape and physical strength, like your skeleton does for you. Unfortunately it can’t be seen using normal microscopy.
We need another clever idea. That’s where labelling comes in. You can add chemicals to the cells that stick to the parts you’re interested in, like the cytoskeleton. These labels can be created by a bit of genetic manipulation. Jellyfish, those floating blobs that can spoil a trip to the seaside, have given science a really useful tool. It turns out that some species of jellyfish ‘glow’, just like false teeth or dandruff do at a disco. You can take the jellyfish genes that make them glow, (called ‘Green Fluorescence Protein’ or ‘GFP’), and add this genetic instruction into other cells so that parts of them glow in the dark when a light is shone at them. You can see where the jellyfish marker is attached and with confocal microscopy take slices through this new luminous label, showing up the parts of the cell you’re interested in.
Actin apart, what’s the bigger picture?
Actin is a part of the cell cytoskeleton that helps give it its shape, and it can be labelled with the GPF jellyfish gene. Being able to see how the shape of the actin in a cell changes under mechanical stresses is a first step to being able to understand how cartilage cells work (or don’t work) properly.
Cells going down the tube
You can get the images, but what do they tell you? In comes the computer science, to help measure how the cell is doing and so understand the way cartilage cell changes. Enter the work of computer scientist and biologist, Bushra Akhtar. She wants to help us find out the answer. She is working towards her PhD developing better ways to produce improved images of cells subjected to mechanical forces, and to create computer software to let us automatically measure these changes. She is exploring the use of a method called ‘Digital Image Correlation’. It’s an image processing method that looks for parts of an image that are the same; we say that same-looking parts are correlated. One use of the technique is to automatically spot suspicious behaviour, such as a passenger leaving a bag on a busy station platform.
By looking at slices produced by confocal microscopy her techniques can compute how the labelled cell parts change over time, extracting precise numbers for how the cell parts move and deform when they are subjected to a mechanical load. Bushra sucks cells into a thin glass tube, called a pipette, and calculates how much different parts of the cell structure change as they are pulled in. This gives useful information on how cells respond to pressures and may in future help us understand mechanotransduction better. That will hopefully lead to new medical treatments: all thanks to a jellyfish, a very small hole in a microscope and some novel computer software.
It could just help shape the future of cells!